Argon gas discharge lamp: Difference between revisions
No edit summary |
|||
Line 11: | Line 11: | ||
The glass pipe provides an electrical isolation between the cathode and the anode, and provides a viewport for the experimenter to observe glow discharge. The anode was connected to the positive terminal of a high-voltage power supply, which can reach upto 760 V. Epoxy was used to connect the pieces and seal the vacuum chamber. A ballast resistor (33 kΩ) was added in series with the gas discharge lamp in order to limit the current. The electrodes were mechanically held using laser-cut acrylic plates fixed to an optical breadboard using brackets and bolts. Multimeters were used to monitor the current and the voltage across the lamp. | The glass pipe provides an electrical isolation between the cathode and the anode, and provides a viewport for the experimenter to observe glow discharge. The anode was connected to the positive terminal of a high-voltage power supply, which can reach upto 760 V. Epoxy was used to connect the pieces and seal the vacuum chamber. A ballast resistor (33 kΩ) was added in series with the gas discharge lamp in order to limit the current. The electrodes were mechanically held using laser-cut acrylic plates fixed to an optical breadboard using brackets and bolts. Multimeters were used to monitor the current and the voltage across the lamp. | ||
Additional | Additional details: | ||
* <b>Electrodes:</b> The electrodes were made by boring a hole through the centre of a cylindrical piece of aluminium(?) using a lathe. By facing the surfaces and cutting out the corners at 45 degrees angle, sharp edges were minimised to reduce charge leakage through corona discharge. | * <b>Electrodes:</b> The electrodes were made by boring a hole through the centre of a cylindrical piece of aluminium(?) using a lathe. By facing the surfaces and cutting out the corners at 45 degrees angle, sharp edges were minimised to reduce charge leakage through corona discharge. | ||
* <b>Glass pipe:</b> The glass pipe was cut from a longer piece of quartz glass pipe. | * <b>Glass pipe:</b> The glass pipe was cut from a longer piece of quartz glass pipe. | ||
* <b>Vacuum fittings:</b> The cathode is connected to a metal pipe attached to a KF blank flange with a hole. The rest of the vacuum fittings are connected via rubber o-rings and clamps. | * <b>Vacuum fittings:</b> The cathode is connected to a metal pipe attached to a KF blank flange with a hole. The rest of the vacuum fittings are connected via rubber o-rings and clamps. | ||
* <b>High-voltage probe:</b> The high-voltage of the anode was measured with a voltage divider (1:1000) probe, connected to a multimeter. | * <b>High-voltage probe:</b> The high-voltage of the anode was measured with a voltage divider (1:1000) probe, connected to a multimeter. | ||
Revision as of 14:43, 28 April 2022
By applying a sufficiently high DC voltage across a gas, the gas atoms/molecules are ionised by the strong electric field. In this project, we construct an Argon-based gas discharge lamp, with adjustable pressure and voltage. The breakdown voltage of Argon gas with respect to pressure changes is observed, and compared with Paschen's law. We also observe changes in the spectroscopic properties of the plasma with varying pressure.
Setup
A small air-tight chamber was evacuated with a rotary vane pump to about Torr (measured with a Pirani gauge). Then, Argon gas was slowly introduced to the chamber via a needle valve, until the chamber reached the pressure of about to Torr. The metallic cylinder that interfaces with the gas lines forms the cathode of the chamber, and is grounded. Another metallic cylinder (anode) was attached to the cathode using a glass pipe.
The glass pipe provides an electrical isolation between the cathode and the anode, and provides a viewport for the experimenter to observe glow discharge. The anode was connected to the positive terminal of a high-voltage power supply, which can reach upto 760 V. Epoxy was used to connect the pieces and seal the vacuum chamber. A ballast resistor (33 kΩ) was added in series with the gas discharge lamp in order to limit the current. The electrodes were mechanically held using laser-cut acrylic plates fixed to an optical breadboard using brackets and bolts. Multimeters were used to monitor the current and the voltage across the lamp.
Additional details:
- Electrodes: The electrodes were made by boring a hole through the centre of a cylindrical piece of aluminium(?) using a lathe. By facing the surfaces and cutting out the corners at 45 degrees angle, sharp edges were minimised to reduce charge leakage through corona discharge.
- Glass pipe: The glass pipe was cut from a longer piece of quartz glass pipe.
- Vacuum fittings: The cathode is connected to a metal pipe attached to a KF blank flange with a hole. The rest of the vacuum fittings are connected via rubber o-rings and clamps.
- High-voltage probe: The high-voltage of the anode was measured with a voltage divider (1:1000) probe, connected to a multimeter.
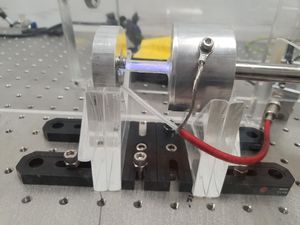
Measurements
The chief advantage of our setup is the capability to adjust the pressure of the Argon gas.
Glow Discharge
We qualitatively observe the changes in the spectroscopic properties in the stable DC Argon plasma, as we vary the pressure. The colours of the glow shifts from pink, to purple, to pale blue, as the pressure increases from approxmiately 0.03 Torr to 3 Torr.
Pressure (Torr) | Video Frame |
---|---|
0.03 | ![]() |
0.3 | ![]() |
3 | ![]() |
File:Argon-lamp-glow-compressed.mp4 (download to view)
Paschen's Law
The ions and free electrons produced from a sufficiently high voltage (above breakdown voltage) move towards the respective electrodes, and current is formed across the lamp. The moving charges collide with other particles to multiply the number of charge carriers in the gas. This process is known as Townsend avalanche or multiplication. The excited atoms and ions decay to the ground state and emit light, in the form of glow discharge.
The change in the breakdown voltage with respect to the pressure of the gas can be described by Paschen's Law, according to which the breakdown voltage given by
where is the pressure, is the distance between the electrodes (here, is constant), is the secondary-electron-emission coefficient, and and are empirically determined constants. represents the saturation ionisation, and is related to the excitation and ionisation energies.
The above graph illustrates qualitatively how the breakdown voltage varies with pressure, under Paschen's law. When the pressure decreases, the mean free path of the moving charges increases, enabling them to accumulate sufficient energy for the multiplication process with a lower required applied voltage. At the same time however, the collision cross-section also decreases, reducing the occurrence of ionising collisions. At a very low , the latter effect dominates, and thus a high voltage is needed for the multiplication to begin.
We were unable to consistently ignite the glow discharge with the high-voltage power supply we had. Initially, this was solved by connecting a laser driver in parallel for ignition as shown.
However, this did not allow us to conveniently measure the voltage across the lamp. We have instead achieved a more consistent ignition without the laser driver, but by momentarily shorting the electrodes with a short piece of wire. The results of the measurements are shown below, with a best-fit line of Paschen's law.
The fit is noticeably bad, with a large deviation for the value of from what we expect. (For Ar, and . [1]) It seems however reasonable to assume that our method of ignition introduces some current through the ballast resistor, causing a non-zero voltage drop across it. With this assumption, we propose a slight modification to the original model:
where a constant voltage translation is introduced to account for the voltage across the ballast. We were able to obtain a better fit as such, with closer values for the coefficients to the expected values.
Hysteresis
Stability?
Summary
References
Source Files
- ↑ Lieberman, M. A., & Lichtenberg, A. J. (2005) Principles of plasma discharges and materials processing. Hoboken, N.J: Wiley-Interscience. 2nd Edition