Schlieren Imaging
Introduction
Schlieren is a method to observe the refractive disturbance of transparent media. It is formally originated by Robert Hooke in the 17th century. At the time it was invented, schlieren was once considered only a qualitative way of visualization. But nowadays, the quantitative usage of schlieren has become widespread in laboratories and industry.
Theory
There exist several kinds of schlieren setup. But the basic idea is to convert the refraction index gradient with a knife-edge or pinhole. Here we only give a representative analysis based on our setup shown below.
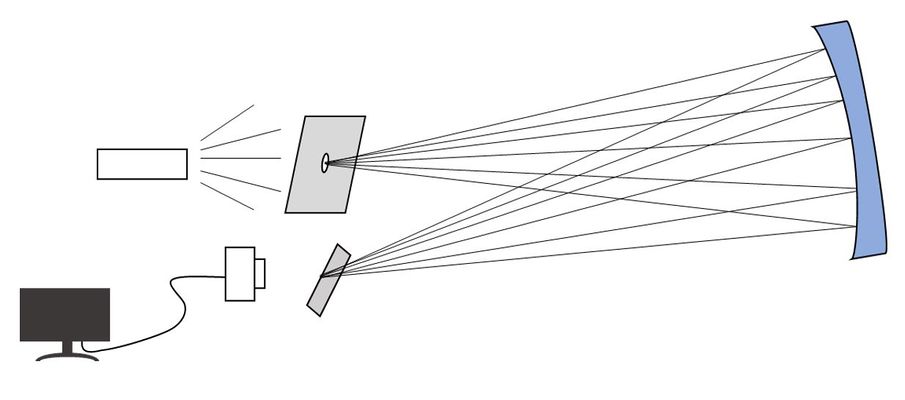
The light coming out from the pinhole is focused by the concave mirror. The position knife-edge is adjusted so that half of the focused light point is cut off. The light field is then recorded by a camera.
When the beam passes through an area with a refractive index, a small deflection occurs. Therefore, whether the corresponding part of the light field can pass the knife-edge gets changed. Only the deflection orthogonal to the knife-edge needs to be considered, which is written as:
In our experiment, the concave mirror is placed from both the light source and the knife-edge. The sample is placed right before the mirror. So the displacement of light on the knife-edge is . From the angle of Fourier Optics, the knife-edge acts as a spatial filtering component, while the concave mirror is a Fourier transformer.
A known density distribution is needed to scale a schlieren setup. We picked an ultrasonic wave for its regular density distribution. A one-dimensional acoustic wave function writes:
The maximum pressure of sound is bounded by the input power . Since the ultrasonic wave has a high frequency of around 40kHz, the state of air could be regarded as adiabatic expansion and compression, which follows . The relationship between the refractive index and the compression of volume fits Gladstone–Dale relation:
where the density of fluid . Therefore, the refractive index distribution caused by an acoustic wave is easily calculated. The thickness and volume of the sound area are fixed by using a standing wave setup. But either a standing wave or a traveling wave causes a fast-changing density oscillation, which flattens the fringes averaged within the time of shutter. Therefore, we used an acousto-optic modulator to produce a flashing light source to phase-lock with the sound wave.
Setup
Version 1
The light source is a high-power LED that drives at about 3V 3A. There is an aperture in front of the led to block most of the light and make the source a point source. A concave mirror is located at 2f from the source. The concave mirror has a small angle to the instance beam. So the reflected light will go beside. There is a knife-edge at the reflection path about 2f from the concave mirror. Its function is to block half of the beam. A camera is located behind the knife edge to view the image.
The picture of the real setup looks like below. The concave mirror is on the left hand of the picture while the camera, knife-edge, and the source are on the right-hand side.
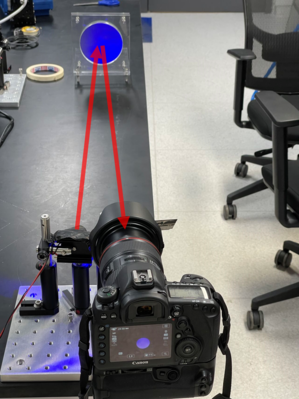
The source and the knife-edge are clearly shown below. A black cover is used to block the stray light so that it will not influence the imaging process as the light from the aperture is relatively too weak. There is a bright blue dot projected on the knife edge. That is half art of the minimum waist radius of the beam. So when the air density changes, the sharp pattern will move up and down and cause the image brighter or darker.
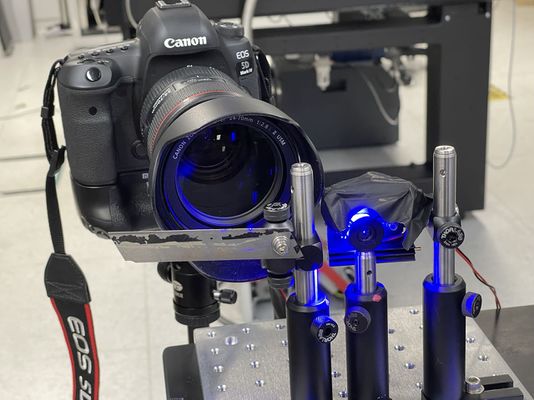
One thing that needs to be careful is not to let the knife-edge/camera be too far from the point source. Otherwise, the smallest wrist will not be round enough. It will come elliptically when the instance beam and the reflected beam are not overlapped very well. The elliptical shape of the beam can harm the resolution of the device.
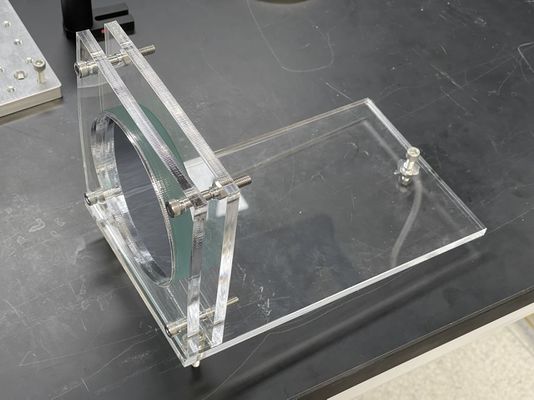
The huge concave mirror is clamped by two pieces of acrylic frame. The frame is pasted on a plate and there are three screws at the bottom of the plate to adjust the orientation and height of the mirror.
Version 2
This is the ultrasound generator. The grey thing is a high-power speaker and the green plate is a circuit board for the speaker. And the speaker is connected to a function generator so the speaker can send sound at the corresponding frequency.
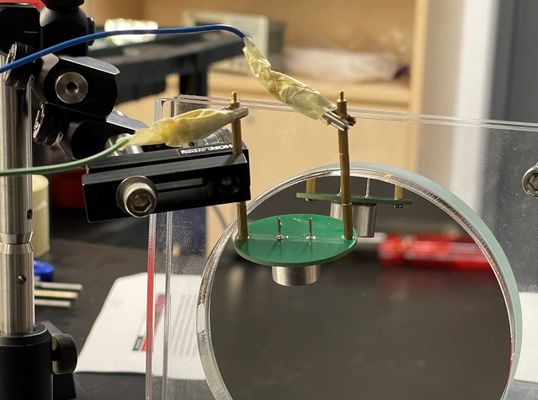
For capturing the sound, we need a high-frequency blinking light. Since the led can not work at 40kHz square wave voltage, we decided to use an independent modulator to shape the light to blinking mode. There is only an Acoustic Optics Modulator in our group at infrared wavelength, we replace the LED with a 650nm laser diode.
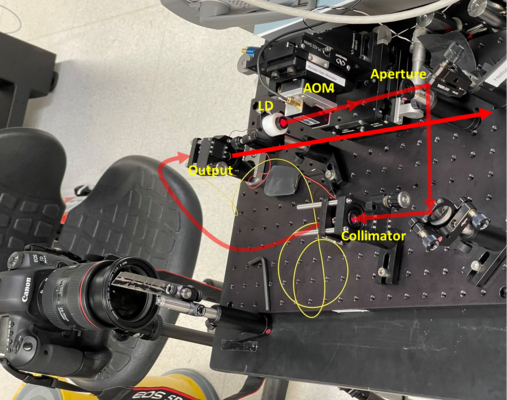
We use a homemade laser driving circuit to supply stable power to the 1mW laser diode. The diagram of the circuit is shown below, thanks to the help from Moe @CQT offering on the design.
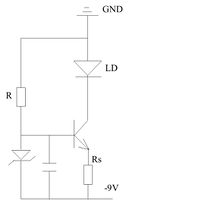
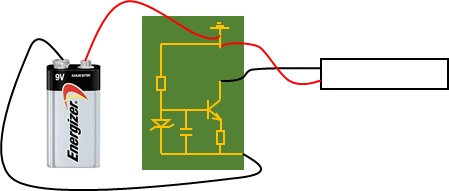
The light first goes through an acoustic modulator to chop the CW laser to a series of pulses. And then, an aperture is used to select the first-order diffraction pattern. Then, two gold mirror is included to adjust the angle and the height of the laser beam. A lens mounted on the cage system can focus the beam on the fiber tip so we can couple the light into a single-mode fiber. After passing through a short single-mode fiber, the output spatial mode will be relatively pure than before.
Two function generators are included, one is for the ultrasound generation. Another is to send a modulation signal to the acoustic optical modulator controller.
Measurements
.... We first capture the airflow of a lighter flame.