Contactless Conductivity Measurement
Introduction
In our Spintronics Lab, when we want to analyze a sample, conductivity or resistivity as a electrical property is almost always necessary. Normally, we use a Voltmeter or standard four probes method to take a measurement. However, surface preparation and contamination have great effects on the electrical properties of the samples. Thus, a contactless technic would be a better way to carry out the conductivity measurement. When we were doing the ac magnetic susceptibility measurement, we learn from literature that there is connection between the electrical conductivity and the ac magnetic susceptibility. We would like to build the set up from the literature, take a measurement on the conductivity of our metal sample and compare it with the result from standard four probes measurement.
Theory
The mechanism for the contactless conductivity measurement is identical to the effective magnetic susceptibility measurement. Basically, the sample is inserted into a primary coil and a secondary coil. An ac current passes through the primary windings will induce magnetic field. The sample responds to the magnetic field and eddy current will be induced within the sample. Then, EMF will be induced in the secondary coil due to not only the driving magnetic field of the primary coil, but also the magnetic field of the currents induced in the sample. Two magnetic fields are 90 degrees out of phase so that the real part and imaginary part of the resultant voltage will be separately related to the ac magnetic susceptibility and the conductivity. When calculating the conductivity from voltage, there are two different approaches introduced by Ishida et al.[1] and Iñiguez et al.[2].
The first approach uses susceptibility to express the magnetic flux penetrating the cylindrical sample and relate the magnetic flux to EMF. It gives rise to the final expressions
which is the real part of the induced voltage and is the imaginary part. , , , ,, are the susceptibility, permeability, conductivity, radius, geometric coefficient and length of the cylindrical sample. , are the frequency and strength of the primary coil magnetic field. Some parameters can be reduced or cancelled out when we divide the two voltages and the equation we eventually use is
The second approach uses............................................................................. It gives rise to the expression
which is the angle between the real and imaginary voltages. D and d are the outer and inner diameter of the sample because this approach chooses tube sample. When we express it in terms of voltages, the expression becomes
To do list
Prepare the samples
Prepare the coils
Build the experimental set up
Measure
Graph and Report
Sample Preparation
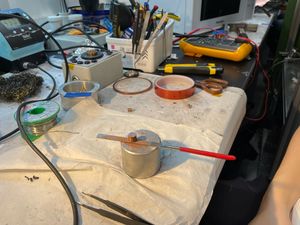
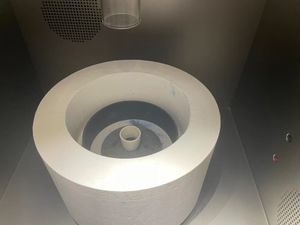
For this experiment, we have tested two samples, copper metal rod (Cu) and sodium doped lanthanum manganite (La1-xNaxMnO3). We choose copper because we have it in the workshop and the theoretical values of some necessary parameters like susceptibility and conductivity of copper are easy to obtain online so that we can calculate and verify our result. We also choose La1-xNaxMnO3 especially La0.95Na0.05MnO3 because we have measured its resistivity value through standard four probes method in our MPT projects. Because of its property of changing Curie temperature with respect to the level of doping, La0.95Na0.05MnO3 at room temperature shows metallic like resistivity behavior.
The copper rod we cut and polished is presented in Figure 1. The radius of the rod is 0.005 meters and the length is 0.0082 meters. The theoretical volume susceptibility we found online is -9.63 * 10-6 and the conductivity is about 58.7 * 106 siemens/meter. We will use these parameters as well as our measured voltage to compute our experimental conductivity.
Experiment Set up
The measuring circuit consists of a detecting coil, a compensating coil with equal turns of copper wire and a primary coil.
Data Analysis
We first measure the copper rod with diameter of 6mm under low frequency range and high frequency range separately both under AC current with amplitude of 1V. The imaginary response in voltage didn't show the quadratic dependence of frequency frequency. Instead both Vp and Vq shows a linear variation with frequency like in Fig.1 which we suppose to be the intrinsic frequency dependence of ac conductivity. As a result the conductivity of Cu rod shown in Fig3 decreases with the increase of frequency which shows a similar value with dc conductivity. around kHz range. In addition, we measured Teflon, an insulator, for comparison.
Conclusion
Team Members
Chen Guohao, Jiang Luwen
Lab Location
Spintronics and Magnetic Materials Lab
References
- ↑ Ishida, T., Monden, K., & Nakada, I. (1986). Electrodeless method for the measurement of ionic conductivity of RbAg4I5. Review of Scientific Instruments, 57(12), 3081-3084. https://doi.org/10.1063/1.1138995
- ↑ Iñiguez, J., & Raposo, V. (2007). Measurement of conductivity in metals: A simple laboratory experiment on induced currents. European Journal of Physics, 28(6), 1125-1129. https://doi.org/10.1088/0143-0807/28/6/009
Bean, C. P., DeBlois, R. W., & Nesbitt, L. B. (1959). Eddy‐Current method for measuring the resistivity of metals. Journal of Applied Physics, 30(12), 1976-1980. https://doi.org/10.1063/1.1735100
Crowley, J. D., & Rabson, T. A. (1976). Contactless method of measuring resistivity. Review of Scientific Instruments, 47(6), 712-715. https://doi.org/10.1063/1.1134714
Oike, H., Miyagawa, K., Kanoda, K., Taniguchi, H., & Murata, K. (2009). Contactless conductivity measurements on the organic conductor, κ-(ET)4Hg2.89Br8, under pressure. Physica. B, Condensed Matter, 404(3-4), 376-378. https://doi.org/10.1016/j.physb.2008.11.023
Kraftmakher, Y. A. (1991). Measurement of electrical resistivity via the effective magnetic susceptibility. Measurement Science & Technology, 2(3), 253-256. https://doi.org/10.1088/0957-0233/2/3/011
Bauhofer, W. (1977). Continuous, contactless measurement of the temperature-dependent electrical resistivity of metals. Journal of Physics. E, Scientific Instruments, 10(12), 1212-1214. https://doi.org/10.1088/0022-3735/10/12/003